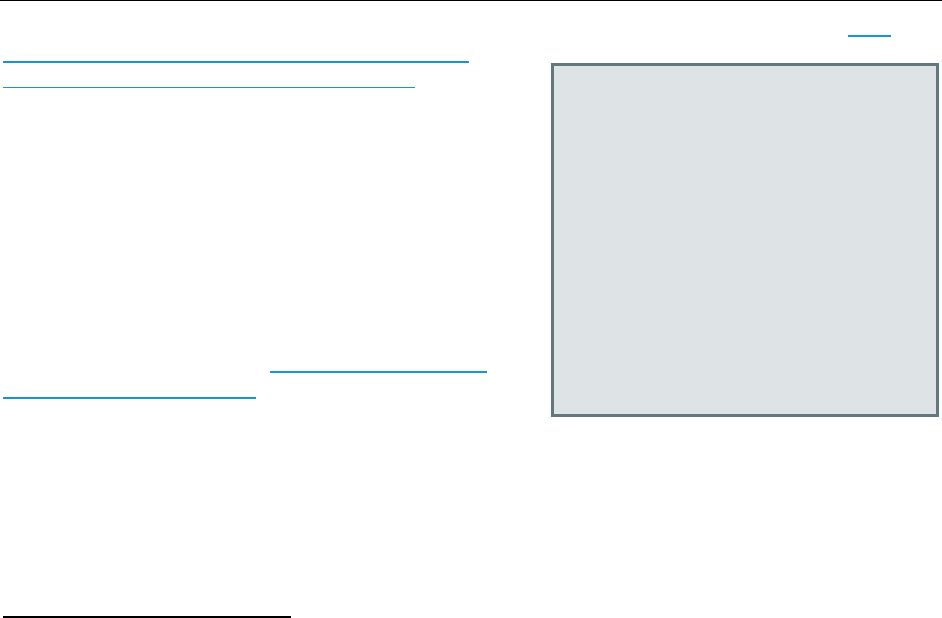
2050 Transmission Study page 8
ISO-NE PUBLIC
Section 1: Study Overview
The New England power system is in the midst of an unprecedented shift in the ways in which
electricity is produced and consumed. Five of the six New England states have committed to
reducing their carbon dioxide emissions by at least 80% by 2050, prompting ongoing changes in
the grid’s resource mix and the increased electrification of the heating and transportation sectors.
1
Driven largely by these statewide commitments, the grid continues its shift toward renewable
resources like wind and solar photovoltaic (PV) generation. Over the next several decades, these
renewable resources are expected to substantially displace natural gas-fired generation as the
region’s primary resource type. At the same time, increased electrification is expected to
significantly increase overall consumer demand for electricity and drive changes in usage patterns
that include seasonal and daily shifts in peak demand.
Among ISO New England’s responsibilities as a Federal Energy Regulatory Commission (FERC)-
authorized Regional Transmission Organization is ensuring the regional power system continues to
operate reliably as system conditions change. Transmission planning helps to maintain system
reliability and enhance the region’s ability to support a robust, competitive wholesale power
market by moving power from various internal and external sources to the region’s load centers.
This 2050 Transmission Study is a pioneering look at the ways in which the transmission system in
New England may be affected by changes to the power grid, and includes roadmaps designed to
assist stakeholders in their efforts to facilitate a smooth, reliable clean energy transition.
1.1 Study Background and Objectives
In October 2020, the New England States Committee on Electricity (NESCOE) released the New
England States’ Vision for a Clean, Affordable, and
Reliable 21st Century Regional Electric Grid. This vision
statement recommended that the ISO work with
stakeholders to conduct a comprehensive long-term
regional transmission study. This study, eventually
titled the 2050 Transmission Study, would help inform
stakeholders of the amount and type of transmission
infrastructure necessary to provide reliable, cost-
effective energy to the region throughout the clean
energy transition.
In response to NESCOE’s vision statement, the ISO
revised Attachment K to the
ISO New England Open
Access Transmission Tariff to incorporate a new
transmission planning process designed to look beyond
the current 10-year planning horizon. The first phase of the effort established the rules that will
allow New England states, through NESCOE, to request that the ISO perform longer-term scenario-
based transmission planning studies, such as this one, on a routine basis. Changes to the ISO Tariff
were approved by FERC in early 2022. The 2050 Transmission Study is the first example of its kind
within New England.
1
The six New England states are Connecticut, Maine, Massachusetts, New Hampshire, Rhode Island, and Vermont. The five
states with the emissions reduction goals described here are Connecticut, Maine, Massachusetts, Rhode Island, and Vermont.
Who is NESCOE? NESCOE is a not-
for-profit entity that represents the
collective perspective of the six New
England Governors in regional
electricity matters and advances
the New England states’ common
interest in the provision of
electricity to consumers at the
lowest possible prices over the long-
term, consistent with maintaining
reliable service and environmental
quality.